CERN70: Tasting the primordial soup
18 October 2024 · Voir en français
Part 19 of the CERN70 feature series. Find out more: cern70.cern
Jürgen Schukraft joined CERN’s heavy-ion programme in 1986 and was the first spokesperson for the ALICE experiment at the Large Hadron Collider
(Image: CERN)
On 7 November 2010, lead nuclei collided in the Large Hadron Collider (LHC) for the first time. In the control room of ALICE, the LHC’s heavy-ion experiment, a celebratory atmosphere reigned. This was the beginning of a new chapter in CERN’s heavy-ion programme, which had started 25 years earlier.
In the 1980s, theoretical work had indicated that high-energy heavy-ion collisions – using, for example, lead or gold nuclei – would allow quark–gluon plasma, a state of matter thought to have existed at the very start of the Universe, to be reproduced. Less than 10 microseconds after the Big Bang, the Universe was too hot and dense for quarks and gluons to bond together to form protons and neutrons, the constituents of atomic nuclei as we know them. Quarks and gluons moved around freely in a sort of primordial soup known as quark–gluon plasma.
In 1986, the programme’s first experiments were carried out using relatively light nuclei, such as oxygen and sulphur nuclei, which were fired by the SPS (Super Proton Synchrotron) accelerator at targets made of heavier elements, such as gold and lead. These experiments collected promising clues, but did not produce conclusive results confirming the existence of the much-talked-about primordial soup. A second programme started in 1994 with lead ion beams. A total of seven large experiments (and other smaller ones) measured different aspects of the collisions. The combined results collected by these experiments allowed CERN to announce the discovery of a “new state of matter” in February 2000. At the same time, the Brookhaven National Laboratory in the United States started up its Relativistic Heavy Ion Collider (RHIC). In 2005, the Brookhaven Laboratory announced that it had observed quark–gluon plasma and its characteristics were unexpected. The plasma behaved like an almost perfect liquid with practically no viscosity.
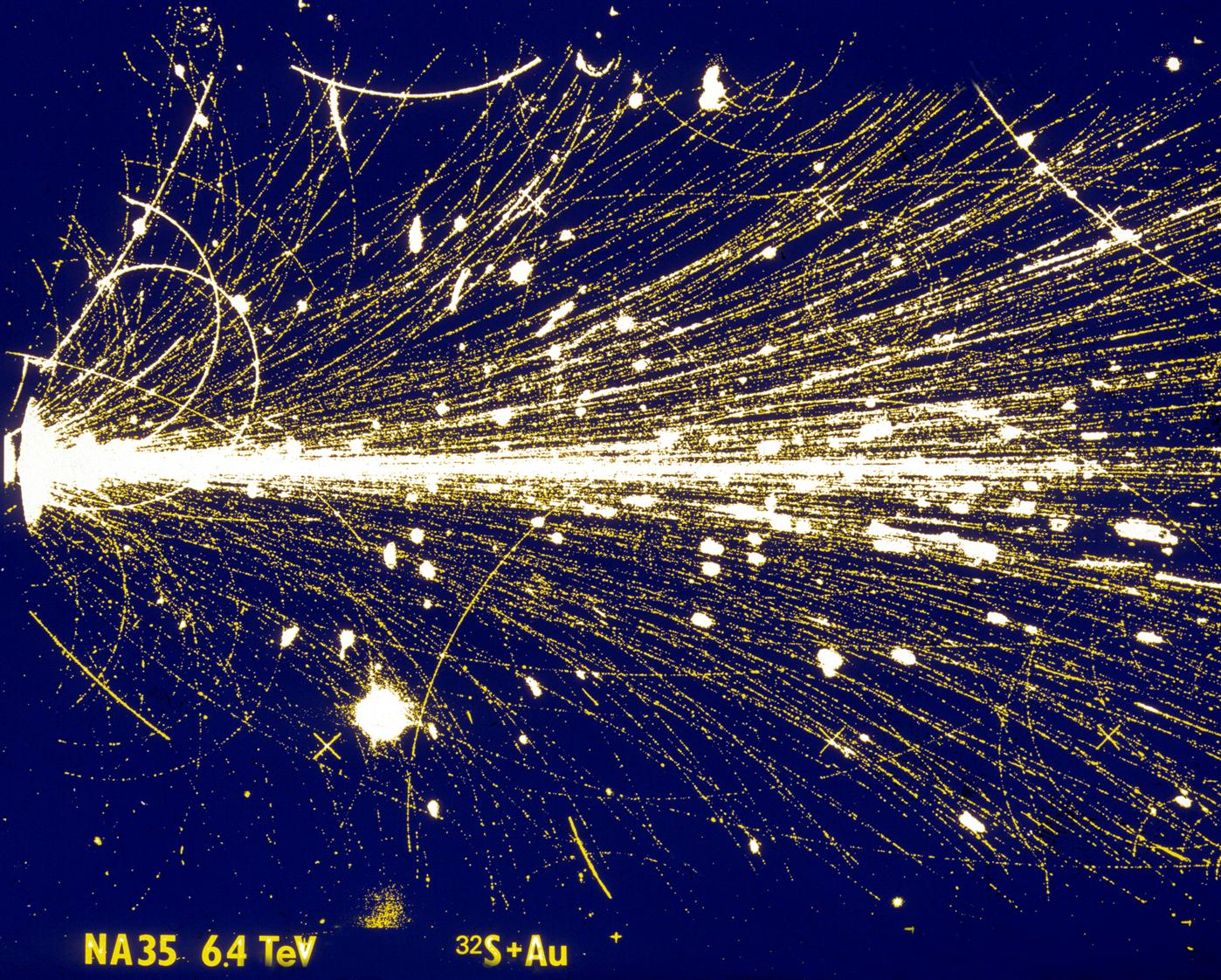
During this time, at CERN, the Large Hadron Collider collaborations were preparing to assemble their detectors. In 1993, a group of scientists led by Jürgen Schukraft had proposed the construction of a detector for the study of heavy-ion collisions, in order to carry on the programme that had started a few years earlier at the SPS. Known as ALICE (A Large Ion Collider Experiment), the experiment was designed to identify the multitude of particles emitted by heavy-ion collisions. With the first proton collisions, which were followed by lead-ion collisions in 2010, ALICE started exploring the strong interaction and quark-gluon plasma.
In 15 years of operation, ALICE, along with the other LHC experiments, has produced a large quantity of detailed results that allow us to better understand the state of matter at the very beginning of the Universe. The experiment has, for example, studied the behaviour of plasma and determined the characteristics of its flow. It has also observed the phenomenon of jet quenching, which reveals the energy loss of quarks and gluons in plasma, and has defined more precisely the production of the various particles in heavy-ion collisions.
Recollections
It took 20 years to build the ALICE detector, 40 minutes to take the first data, one hour for preliminary analysis, two days for the (almost) final results, and three days to agree on the author list. The ship had set sail, and the physics journey into ALICE’s wonderland had begun in earnest.
Jürgen Schukraft
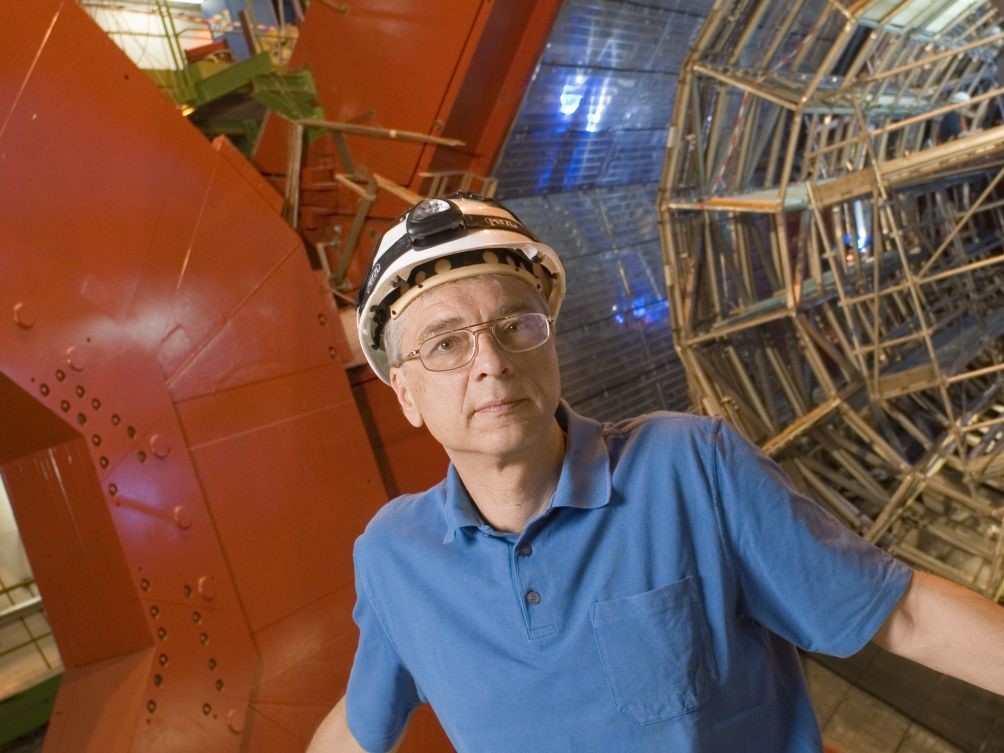
After two years at the Intersecting Storage Rings (ISR), Jürgen Schukraft joined CERN’s heavy-ion programme when it started data taking in 1986. He worked on the North Area experiments NA34 and NA45 before starting at ALICE, CERN’s heavy-ion experiment at the Large Hadron Collider (LHC), for which he was the first spokesperson from 1993 to 2011.
“The beginning of the heavy-ion programme at CERN in the 1980s was both exciting and confusing. We made a big leap in energy with SPS and thought that it might be enough to create a quark–gluon plasma (QGP). Everyone was in a “gold rush” mood, but nobody knew what we would find or when. During the programme’s first days, Austrian TV came to CERN wanting to film the “first detection of the quark–gluon plasma” live. They were disappointed when they realised that it would take more time...
Even though we found two striking signatures that were predicted for a QGP, the first results were confusing. Better theory was needed, and more data. From 1994, we could use heavy ions (lead), which made all the difference. Plus, a new generation of bespoke detectors opened new possibilities, and evidence that we were looking at something “different” was accumulating. In early 2000, CERN announced “compelling evidence” for a new state of matter. Yet, we still couldn’t be sure if this “new state” was the real QGP or only a precursor, or even an imposter.
The centre of action moved to Brookhaven National Lab with the startup of RHIC in summer 2000. By 2005, BNL declared that yet another new state of matter had been found – a “strongly interacting perfect liquid” – an even more fluid substance (with less internal friction) than superfluid helium. Around the same time, the SPS confirmed another crucial prediction, the “melting” of hadron masses in the QGP and also precisely measured the QGP temperature, far above the predicted threshold. By then we knew, in hindsight, that we had been dealing with the QGP all along.
Back at CERN, we were busy inventing new detector technologies for the LHC, building and assembling the (for us) gigantic ALICE experiment, and writing thousands of pages of technical documents. After an aborted attempt the year before, the LHC saw “first light” during a short proton–proton test run in November 2009. The packed ALICE control room erupted in applause when the first collision “event” popped up on the screen. The display looked good, the detectors worked and decades of preparation had come to a successful end.
A young fellow sitting quietly in the back of the room had single-handedly reconstructed and analysed the 284 (!) events that we netted during the test. Within an hour, he interrupted our celebration with the first actual physics result, the density of produced particles. It looked good, and the next morning, literally in the shower, I had the crazy idea to see if we could push this all the way to publication. The following days were the most intense and exhilarating of my life. The detector had worked out of the box, but of course not perfectly; in intense meetings twice a day, with a 24-hour task force of a few dozen people, we sorted out one problem after another. By day five, one minute after midnight, the paper was submitted – a full week before the first scheduled LHC collisions. It took 20 years to build the ALICE detector, 40 minutes to take the first data, one hour for preliminary analysis, two days for the (almost) final results, and three days to agree on the author list. The ship had set sail, and the physics journey into ALICE’s wonderland had begun in earnest.
And what an expedition it was. In the years since, the LHC heavy-ion programme has helped to resolve most of the earlier puzzles. Notably, we understood the confusing ups and downs of charmonium suppression as an unanticipated consequence of the QGP. With heavy-ion results also coming in from the other three large LHC experiments, we measured the properties of the QGP with ever-increasing sophistication and precision. And early on, still with proton beams, we tripped over another surprise – the first, and arguably still the most unexpected, LHC discovery. A feeble, but distinct, very long-range correlation between particles, never before seen in any elementary collisions, was announced in 2010 to a packed auditorium by the CMS Spokesperson with an apology (“we present this signal to the scrutiny of the scientific community because we didn’t succeed in killing it”). The mysterious result spawned many different explanations, which were mostly ad hoc, speculative, or outright weird. Today, after a plethora of painstaking and detailed measurements, including crucially in proton–nucleus collisions, and alongside great strides in theory, we understand this correlation – unexpectedly appearing in “lowly” proton–proton collisions – as a precursor or onset of the hot and dense matter phenomena we had considered the exclusive territory of heavy-ion reactions.
Forty years after seeing (or rather, understanding) not much in those first light-ion collisions at the SPS, we are now regularly exploring and testing with remarkable detail and precision QCD (quantum chromodynamics), the theory of the strong interaction, in the regime where it actually is strong. Along the way, we have found traces and shadows of the QGP everywhere we looked, including in the lightest of systems. Such are the surprises and adventures of exploring nature at the frontiers of our understanding, and it has been a privilege – and lots of fun – to be part of it for so long.”
----
This interview is adapted from the 2004 book “Infinitely CERN”, published to celebrate CERN’s 50th anniversary, updated by Jürgen Schukraft in 2024.